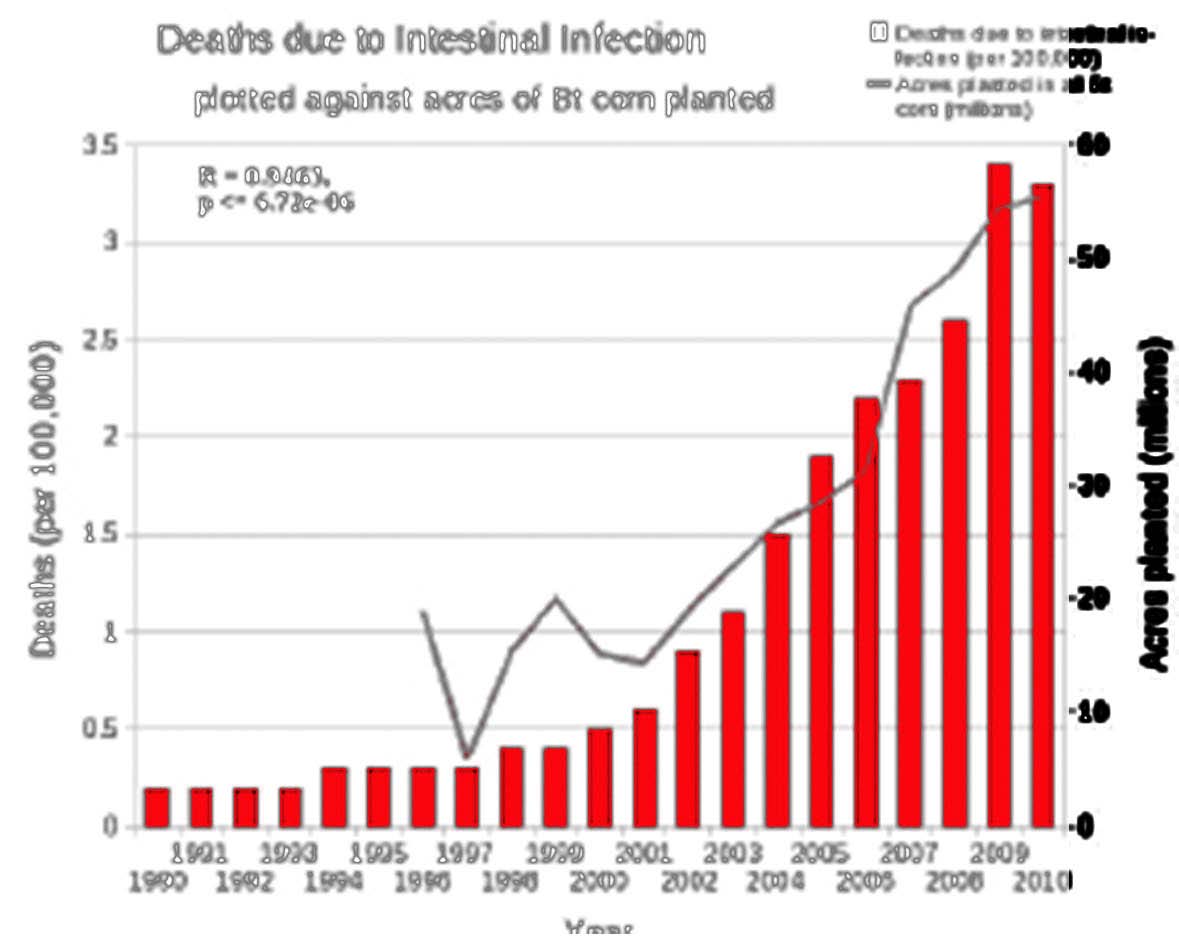
hello everyone. i’d like to thank you forjoining us for this webinar. my name is erica qiao, i’m with bcs, incorporated. i currentlyprovide support to the bioenergy technologies office, and will be moderating the webinartoday. to begin this webinar, i will provide a brief overview of the bioenergy technologiesoffice, which will be followed by a presentation from dr. richard sayre, representing los alamosnational laboratory and the new mexico consortium.please record any questions and comment you may haveduring the webinar and send them to eere_biomass@ee.doe.gov. as a follow-up to the webinar, the presenter(s)will provide responses to selected questions.all slides from this presentation will be postedonline at the following web address. for general questions regarding the bioenergy technologiesoffice, please email eere_biomass@ee.doe.gov
o as some additional background on today’swebinar, i’d like to remind everyone that this is part of the bioenergy technologiesoffice’s ongoing webinar series. our webinars cover many of the office’s activities andfeature "hot topic" discussions that are relevant to the development of renewable fuels, power,and products from biomass resources.more information can be found on our website, here. o to providea bit more information about the bioenergy technologies office, we’ve outlined somekey points here on our vision, mission, and strategic goals, which include: helping totransform the nation's renewable and abundant biomass resources into cost-competitive, high-performancebiofuels, bioproducts, and biopower. beto supports ongoing research and developmentefforts to help create a robust, next-generation
domestic bioenergy industry in the unitedstates. and by doing this, the department of energy supports the effort to provide americanswith sustainable, renewable energy alternatives. these efforts enhance u.s. energy security,reduce our dependence on oil, provide environmental benefits (including reduced greenhouse gasemissions), and create economic opportunities across the nation. beto’s mission is tosupport research, development, demonstration, and deployment of advanced bioenergy. ouroffice spans the bioenergy supply chain. feedstock supply efforts focus on rd&d todevelop cost-effective integrated logistics systems—so this includes growing, harvesting,collecting, storing, preprocessing, handling, and transporting quality feedstock to biorefineries.our conversion r&d program is focused on developing
commercially viable technologies to convertterrestrial and algal feedstocks into liquid fuels, as well as bioproducts and biopower.the demonstration and deployment (d&d) program is focused on demonstrating and validatingbiomass conversion technologies through successful construction and operation of cost-sharedpilot, demonstration, and commercial scale integrated biorefineries.we have crosscuttingprograms in sustainability and strategic analysis. proactively addresses sustainability of advancedbioenergy to enhance its benefits, public acceptance, and long-term viability. so, now,i’d like to turn the floor over to richard sayre, of los alamos national laboratory andthe new mexico consortium to provide his portion of today’s presentation on the geneticallymodified algae: a risk-benefit assessment.
hello, i’m richard sayre, i’m a seniorresearch scientist and los alamos national laboratory, and it’s my pleasure today topresent some of the work we’ve been focusing on, addressing the risk and benefit for geneticallymodified algae for producing biofuels, as well as high value coproducts. on this nextslide, is an outline of the presentation that i’ll be giving today. we’ll start witha historical perspective, and that focuses on how has gm traits been managed in the agriculturalsector. what are some of the known potential benefits and risks of gm traits in crops,and how are those agricultural traits managed. then let’s shift to the special case ofalgae, and we’ll start with what is the potential for algae in meeting renewable fuelrequirements, what is the role of gm traits
in producing biomass, what re the perceivedrisks for gm algae, how can those risks be mitigated, what agencies are currently regulatingthe release and commercialization of gm algae and then finally i’ll present some examplesof emerging gm traits from our group that focusing on crop improvement, and then finallysummarize the presentation. so on the next slide, we are looking some of the historicalissues surrounding gm plants for agriculture. and the primary driver that has really pushedthis industry is increased income generation, and as you can see the increase in crop valuefrom genetically modified traits has increased by 98 billion dollars since their initialrelease since 1996 to 201. gm technology, importantly, is also a part of the sustainablesolution for agriculture, and as a result
of the adoption of gm crops, there have beensubstantial reduction in co2 emissions as well as in pesticide use. a large part ofthis reduction in co2 emission and also pesticide use is associated with the ability to do no-tillagriculture. and no till agriculture, particularly in soy beans, was really made feasible bythe introduction of herbicide resistant traits. very importantly, in the us, it’s been estimatedthat over a trillion meals of gm foods have been served since 1996, and to date thereare no known illnesses that have been demonstrated form the consumption of gm foods. so there’san incredibly strong safety record for the management and utilization of gm foods, inaddition there’s been increasing international adoption of gm crops. and most importantlyin developing countries, and in fact in the
last two years, the acreage in gm crop indeveloping countries has exceeded that of developed world, and it continues to growat a relative high rate, at about 6% increase in acreage per year. however, we face someemerging challenges, particularly with respect to global food security, and it’s in thiscontext in which gm technology perhaps will have its greatest benefits. i’m going togive two examples that support this position, the first being the c4 rice project, that’sfunded by the bill and melinda gates foundation. this project is managed at the internationalrice research institute in the philippines. and as a result of the work that they do atirri, analyzing the natural diversity within their vast collection of rice varieties, aswell as wild relatives, they’ve come to
the conclusion that by 2050, the genetic variationthat can be utilized for crop improvement will be exhausted. and so to continue to theprovide food to the roughly 1/3 of the population that consumes rice, they really need a quantumleap in crop production and that’s led to the funding of the c4 rice project. the objectiveof that project is to engineer a c3 low efficacy rice crop into a high efficiency c4 rice crop.closer to home, the biocassava plus project, also funded by the gate foundation, and forwhich i was the principal investigator, actually came to the conclusion that the bioforitfiedcassava, to meet the minimal iron requirements in a cassava based diet, could only be achievedby genetic modification. the genetic variation for iron content in cassava roots was insufficientto hit our target value, and this conclusion
was actually reached by another project fundedby the gates foundation called harvest plus. so there’s opportunities for gm technologyto address major challenges facing the future for food security. so if we look at some ofthe drivers and benefits for transgenic crops in the us, as i mentioned earlier, incomegeneration is probably the most significant driver, and that’s due to the lower costof growing gm crops compared to non- gm crops. in addition we have more sustainability growingmany of the gm crops relative to non-gm crops associated with reduced energy usage and no-tillagriculture, and the reduced use of broad spectrum synthetic pesticides. associatedwith the use of no-till agriculture is of course the reduction of soil erosion. andso again, in many cases, gm crops can actually
provide increased sustainability relativeto non-gm crop, but in the future, particularly in the developing countries, we will see applicationof gm technology to provide better nutritional composition of food extend shelf life of foodsso that they can be marketed into urban areas, increased stress tolerance, particularly droughtand heat tolerance, which are emerging considerations associated with global climate change. theproduction of renewable green chemical feedstock, including biofuels and perhaps pharmaceuticalsin pathogen free organisms that don’t support the growth of pathogens that can infect humans.so of course there are risks associated with any technology, and it’s the balance ofthe benefits and the risks that help us decide whether the technology should be put forwardor not. with respect to transgenic, one of
the challenges that we face with the introductionof the transgene into the plant and also in algae in most cases is the random event, sothere may be unintended mutations associated with the introduction of the transgene thatare potentially undesirable, fortunately, in most cases those are readily identifiable,particularly to genome sequencing approaches, but also phenotypic analysis. another concernis, and a serious concern, is transgene escape to related plants and pollination to nearbyrelatives. in many cases, this issue is managed by limiting the release of transgenetic cropsonly to those areas where progenitor species are not native, but in some cases that's notpossible, and for example herbicide resistant genes moving from canola, an oilseed crop,into other related weedy species, resulting
in herbicide resistance in those weedy species.another concern is increased use of herbicide. so one of the predominant traits in gm agricultureis herbicide resistance to control weeds and associated with that is the increased useof herbicide. so ideally, herbicides used for weed control should be of low toxicity.and then, as i mentioned earlier, the development of herbicide resistant weedy plants. anotherconcern that’s been expresses, particularly by the non-scientific community, but alsowithin the scientific community, is the concern that genetically uniform strains being plantedas a result of the introduction of gm traits. now those two events are not linked necessarily.though the introduction of gm traits does not have to be associated with geneticallyuniform crop, but of course there are concerns
about using the uniform crops, particularlywith respect to disease and stress. there are also unanticipated alterations in foodcomposition, and this really gets back to the first point, and there are the potentialnew mutations, perhaps in pathways that are critical to the production of molecules thatare important in nutrition and food consumption. but another concern is that there may be alterationin metabolic flow associated with genes that are unintended. to address that concern, typicallywhat is done is compare the composition of metabolites of gm foods to non-gm foods tosee if there are alterations in the composition. another concern is the expression of new allergens.and so, there have been examples of strategies for introducing proteins have enriched compositionsof essential amino acids that potentially
come from allergenic proteins. fortunatelyin the review process, these issues are recognized and there are now standard approaches usingbioinformatics tools to identify potential allergenic domains and identify primary proteinsequences. there are also downstream problems, and that is primarily the segregation of transgenicfrom non-transgenic crops. and so, particularly in the european community, they're regulationsabout the potential contamination of non-transgenic crops with transgenic crops. and so that introducesand important step in the growing, processes and distributing these crops, and that isthe demonstration that non-transgenic crops are not contaminated with transgenic crops.and because of the high cost of commercializing transgenic crops, there tends to be greatermarkets controlled by fewer and fewer producers.
so to manage these risks and benefits in theus there are a number of regulatory agencies that can be involved in different stages inthe approval process for the testing and commercialization of gm crops. the examples that i'm going togive here are some of the issues that we had to address in the biocassava plus program,to not only conduct field trials in the us, but in africa, under the most stringent requirementswith regard to the cartenga protocol. so primarily, the first issues we need to address with transgenicsin the us is the issues address in the plant protection act, and that is no sequences canbe introduced from pathogens of human origin into plant materials. generally, that is nota challenge. the next issue is transgene products potentially toxic or allergenic, and so theenzymes, for example, that may be the product
of a transgene produce a toxic molecule orcould itself be allergenic. and as i mentioned earlier, there are bioinformatics stragglesthat could help address those concerns. another aspect is preventing the introduction of nonessentialdna sequences into transgenic plants. so only those sequences involving the expression oftransgene should ideally be introduced into the transgenic plant. which as i mention earlierthe integration of the transgene into the plant genome should have no off target effectand yield a nutritional composition, unless it’s enhanced, in the transgenic plant,should really be identical or have no substantive alteration relative to wild type plants. thenext state is the conduction of confined field trials. these are generally conducted undernationally or internationally recognized protocols.
some of the examples of those safety concernsthat we had to address regarding the cartagena protocols include fencing off the field trialareas, providing continuous 24 hour surveillance, and leaving the fields to lie fallow for atleast a year after the trial is finished to ensure that no volunteer organisms or transgenicsreemerged after the field trial had finished. all flowers have to be systematically removed,so that the possibility of pollen seen dispersal is greatly diminished. and animals that potentiallycould disseminate seeds or portions of the transgenic crops also have to be restrictedfrom the field crop trial area. finally, at the end of the field crop trial, all the materialsmust be destroyed. and typically, that's done by burning all of the residue in a pit, followedby covering that material with soil. so after
the field trials are carried out, ideallythe results should so no consequential or unintended impacts on yield, and as i mentioned,or on the environment. the next stage is taking the food materials, if this is a food, andconducting animal feeding trials to demonstrate that there are no adverse effects on animal’snutrition or health. if those feeds or food materials are ultimately consumed by humans,then the next stage is to demonstrate the complete digestion of transgenic protein inan artificial human stomach, and that no segments of the protein remain undigested after thistreatment. after positive results from in-vitro trials, then it moves onto human feeding trials,again, ideally showing no adverse effects on health or nutrition, and finally regulatoryreview and approval. in total, this is a well-developed
process that has served the transgenic cropcommunity very well. so i’m switching gears and now focusing on algae. the reason thatwe're particularly interested in algae is of course their ability to produce tremendousamounts of biomass in a very small area, and it's been estimated and in fact demonstratedin a variety of trials that algae potentially can produce 2 to 10 fold more biomass perunit per year than terrestrial crop, and so we're looking at exploiting that very highproductivity and rapid growth to produce renewable fuels and also high value coproducts. so asindicated here, algae have high photosynthetic efficient, every cell in microalgae is photosynthetic,unlike other plants that have roots and stems and other tissue that may not be photosyntheticand have a photosynthetic demand. they're
capable of very rapid division, and importantlythey can have high lipid content, as shown in the figure. in the figure, the lipid dropletsare visualized using a florescent dye that is shown here as yellow fluorescent. importantly,we can also recover all the biomass when harvesting, we're not leaving behind residues in the fields,as is the case which terrestrial crops, and we potentially can harvest algae throughoutthe week, through the day, and perhaps throughout the year. this is important because it substantiallyreduces risk, so in terrestrial crops, there is the possibility of having crashes thatcan be associated with adverse weather or disease, for example, and then one may haveto wait a year before another crop is grown. in our experience in cultivating algae thatwe have crashes, generally these can be mitigated
very rapidly, and a new production systemcan be put on line in a matter of days. and, very importantly, algae are perhaps more sustainablemeans of producing biofuels than terrestrial crops, and the part this is associated withthe ability of algae to capture co2 in ponds as bicarbonate. so co2 generated at pointsources can be injected into ponds, hydrated to form bicarbonate, which is non-gaseous,and bicarbonate is then actively pumped into the algae, which is used as a substrate forphotosynthesis. in addition, we can use wastewater to provide nutrients and there's no directcompetition with food if we use or sites to grow the algae in non-agricultural areas.so why is gm technology being considered as part of the solution for algal crop improvement?in part, this is because at the present time
we don't have breeding systems in place forcommercial algal strains. chlamydomonas, which is more or less the lab rat of algal moleculartechnology, the dominant generation is haploid and it is possible to do mating and look atgene segregation and actually look to breed for improved phenotypes. but those breedingsystems have not been developed for most commercial strains at the present time. and so we'releft with other strategies to try to improve the biology and the productivity of algae.those include bioprospecting efforts to identify better strains in the wild than current commercialstrains, using mutagenesis approaches generate new phenotypes that are of high values, andthe introduction of gm traits. all three of these are perhaps our best strategies currentlyavailable for improving the biology and the
performance of algae. in addition, gm traitsand gm strategies can be used to introduce new biochemistries and physiologies in thealgae, for example to produce high value coproducts in high volumes, and this can help substantiallyreduce the cost of producing fuels. and then finally, gm algae, it is possible to growthem in contained fermentation systems to reduce the chance of escape, and so biocontainmentof gm algae is there for feasible, unlike terrestrial plants. so there are a numberof potential risks of course associated with the introduction of gm traits into algae.some of these risks are actually unique to algae and are different from that risk weneed to consider with respect to terrestrial crop plants. one of those risks is aerosolizationand the global spread of algae. being very
small microorganisms on the order of a fewmicrons, they’re very easily suspended into the atmosphere during aerosolization, andin fact it’s known that algae sometime travel great distances across the globe through thejet stream. another concern is that they’re persistent. many algae can survive very longperiods of time completely desiccated in soils and then remerge once water is available.another concern is weedy or invasive traits. if we introduce traits to algae that enhancetheir growth and competition with other algae in the wild, if they have increased abilityto take up nutrients and outcompete other wild algal organisms, if they produce toxinsor express molecules that express evolutionary or competitive fitness, then we have the potentialfor algal blooms of gm algae that may alter
species composition in the environment. andof course there’s always the concern of gene escape to related species in the community.another issue in common with agricultural gm risks is the issue of health impacts. andso it’s generally accepted in the algal community that antigenic proteins will bemanaged as well as limiting the production of toxins. and finally there’s the concernfor mitigating the potential for horizontal or sexual transfer of transgenes. this isparticularly a concern in cyanobacteria which are known to be promiscuous in terms of theirgene exchange capability. so what are some of the potential environmental outcomes? soin this particular figure in the upper left box is a distribution of algal species asindicated in the different colored lines.
so in a natural community there’s oftena diversity of algal communities growing in concert. in the upper right corner is examplesof what we would ideally like to have in a pond, and that is with a gm organism. a monoculture,essentially, that’s highly productive, and high yielding gm algae, either producing moleculesfor biofuels or high value coproducts. if of course we have escape from that pond, inthe lower left box we see a situation where there’s no consequence of the gm algae escaping,in which the species composition is not altered by the release and the growth of the gm organism,and that’s indicated by the yellow line in the lower left corner of the box. you cansee that the population dynamics of the biomass of the gm algae is transient, and does notpersist or alter the species composition in
the wild. the worst case scenario is the lowerright box. in this case the gm algae has increased environmental fitness relative to the naturalpopulation and dominates the species diversity in that environment and perhaps could leadto harmful algal blooms if sufficient nutrients were available to allow it to grow very rapidly.so that’s the situation that of course that we want to avoid from happening with the appropriateregulatory constraints. so ideally gm traits should have a minimal impact on the environment,they should reduce the evolutionary fitness of the algae in the wild, so that they’renot strong competitors with wild algal species, as i mentioned earlier the algae should notproduce toxins or have traits that could be harmful to living organisms, or disrupt ecosystemhealth. it’s critical we evaluate the risk
potential, since in many cases we cannot predictthe outcome of gm traits in the wild, and to do so requires controlled field trialswith multispecies components and these need to be carried out to evaluate the potentialfor risk. and the finally, another strategy is to introduce biocontainment traits intogm algae to reduce their potential for gene transfer. some examples of those strategiesinclude conditional lethality traits that are perhaps complimented by exogenous moleculesin a pond, the inactivation of genes that control sexual transmission to reduce thepotential for gene transfer, and the expression of terminator genes that are activated uponescape from a controlled environmental growth situation. so with respect to the latter traits,what we’re showing here is results from
our group to introduce biocontainment traitsinto gm algae. in this case, the gene that we’re introducing is a gene called barnase,and barnase is a general ribonuclease that degrade rna within the cell. now fortunatelybarnase can be inhibited by a protein called barstar, which has a very high affinity forbarnase. and barstar, in turn, in our case is going to be regulated under the controlof a gene switch. so this gene switch in turn is regulated by caffeine. so in the contextof this application for growing algae in the presence of caffeine, and under those conditionsbarstar gene is expressed and inhibits the barnase ribonuclease activity. when caffeineis not present, of example if the algae were to escape a pond that contained caffeine andgo to an environment where there is no caffeine,
then the barstar gene is inactivated, andthe barnase activity in turn takes over and limits the growth of the cells. so we’vehad good success with this technology, we’re getting substantial suppressions and growthin the absence of caffeine, and we’re now modifying that to eventually show no growthin the absence of caffeine. so it’s this kind of approach, potentially using terminatorgene technology, that’s regulated by a xenobiotic that could only be found in a pond environmentthat may allow us to contain gm organisms. so moving on, i want to briefly address someof the regulatory environment regarding the release and commercialization of gm algae.i’d also like to direct your attention to the bottom of the slide, for a slide showthat was presented by dr. david glass at the
recent algal biofuels biomass and bioproductsconference in santa fe in 2013. and in david’s presentation, he goes into a thorough coverageof the regulatory environment of gm algae. so very briefly, gm algae is regulated bythe epa under the toxic substance control act, or tsca, and if an organization anticipatesdoing a field trial or an outdoor release, the process involves making an applicationto the epa under the tsca experimental release application process. that undergoes a reviewand hopefully an approval, and that takes about 60 days advanced notice for that processto work through. for commercial activity there’s another approval process called the microbialcommercial activity notice (mcan) and that requires and additional period of notificationfor epa review and approval. in addition,
other regulatory agencies besides the epa,including usda and fda may have regulatory approval required for the release or commercializationof gm algae and that will depend on the types of products that are produced and their particularuse, and again i refer you to the presentation by david glass. so finally, i want to mentionsome of the work that we’re doing on algal biofuels, and this is more or less, on thisslide is a summary of lifetime techno-economic analysis that was carried about by the nationalalliance for algal biofuels and bioproducts. that program began in 2010, and finished in2013, so in 2010, we have a base case scenario where algae generic were being grown in openopens, they were harvested in centrifugation, they were typically dried and wet solventextracted, there was no nutrient recycling,
and the oil was converted typically dieselby transesterification. so we modeled that base case scenario as part of the nabb programfor a large-scale production facility in pecos, tx, and the estimated cost of producing fuelfrom that base case scenario ranged from $240-$16, so non-competitive with petroleum at thattime. after 3 years and the integration of the best technology we could identify throughthe nabb program, we’re now approaching $8/gallon. still higher than $3 a gallon forgasoline gallon equivalents (gge) from petroleum, but a substantial improvement over the basecase scenario in 2010. the two factors that are going to have the highest impact thoughon reducing the cost in the future were also identified, and that is productivity of thealgae and the cost associated with harvesting
those algae. to move the price from $8 to$3 will require at least a threefold increase in productivity over the generic or base casescenario in 2010, and also a substantial reduction in the harvesting cost of producing thosealgae. and this is the case where gm can have a substantial impact, by increasing the yieldof productivity of the algae. so in our group, we’ve been trying got prove the biomassproductivity of the algae by increasing the apparent efficiency of photosynthesis. andon this figure that was drawn from a review article by zhu, et al, one can derive thevarious inefficiencies in photosynthesis into three major problem areas. the first beinglight captures, the second is the energy conversion, or biochemistry, and the third factor is energyaccumulation. and it's apparent from this
diagram that most of the losses in efficiencyactually offer in the light capture, and it's conversion into charge separated stage. theoverall efficiency of photosynthesis in crop plants however is very low, ranging from 4-6%on this particular figure but often times in the field it's even less than that, inthe range of 1-3%. so there's substantial room for improvement, theoretically, basedon thermodynamic evaluation, it's potentially feasible to increase the efficiency up to11% using solar energy. if we use the energy of red photons it's even higher, sometimesup to 30% so there's substantial room for improvement in photosynthetic efficiency.so our strategy was really to address the constraints around light capture and energyconversion in the photosynthetic electron
transfer constant. and as indicated by thered here, the maximum rate of photon capture at full sunlight are actually 10 times fasterthan the maximum rates of election transfer. and that's due to the fact that there's akinetic bottleneck between photo system two on the left and photo system 1 on the right,which is the cytochrome b6 complex. and so this is the slowest step in the electron transferprocess and has a rate constant ranging from 1-10 milliseconds. so the rate of photon captureis 10 times faster than the rate of election transfer through the cytochrome b6f complex.so our strategy to address this challenge of the kinetic bottleneck in election transferwas not to improve the efficiency of the election transfer through the cytochrome b6f but toactually reduce the efficiency light capture
to better couple the kinetics of light captureby the light harvesting antennae complex to the downstream kinetic bottleneck in the electiontransfer. so on the left side of the diagram is shown the typical architecture of lightharvesting antennae and photosynthetic complexes of plants in the canopy architecture or inalgae as they grow in a pond. and that is all of these leaves, for example in the canopyarchitecture, or the individual algal cells have very large antennae, and so the leavesor the algae at the top of the canopy or the bond are capturing full sunlight and see manymore photons than they can actually convert into charged separated states, and so up to90% of energy that’s captured by the antennae is actually dissipated as heat or fluorescence.and of course the light intensity is diminished
as one goes down in the canopy and some pointthe antennae are actually too small to capture photons to carry out photosynthesis. now thisparticular antennae architecture actually works very well in mixed species environments,were light is actually a resource that needs to be captured more efficiently by any givenspecies than your competing species. otherwise you're likely to lose in that competition.so having large but inefficient antennae actually provides the selective advantage because theorganism can restrict access to other competing organisms to light. now in a pond environment,ideally we like to capture every photon and use every photon to do photochemistry andto drive photosynthesis. now on the right side is a different type of architecture forantennas. and in this architecture we have
smaller antennas and if it's a fixed canopyarchitecture such as in a forest, smaller antennas at the top of the forest, and largerantennas at the bottom, and so less energy is lost, more energy penetrates deeper intothe canopy or the pond and can ultimately be captured by the antennas than algae orleaves lower in the canopy. to test this hypothesis that smaller antenna may actually increasephotosynthetic efficiency we decreased the size of the antenna in transgenic algae byinhibiting the production of chlorophyll b. chlorophyll b is one of 2 species of chlorophyllfound in high plants and green or algae, and chlorophyll b's function is only to servein the antenna. chlorophyll a is also found in the antenna, but also in the reaction centerswhere charge separation occurs. and so by
limiting the production of chlorophyll b specificallywe can actually reduce the size of the antenna without directly impacting the reaction centerwhere charge separation occurs. and what we demonstrate in this particular diagram isthe rate of photosynthesis as function of the size of the antenna. and here we’remeasuring the size of the antenna by the chlorophyll a/b ratio, so as we reduce the amounts ofchlorophyll b, a/b ratio increases. in this case we see two algae with intermediate sizeantenna, having chlorophyll a/b ratios of a roughly 5 and 4, an algae that has no chlorophyllb is called cbs3, which has a very small antenna, and then wild type algae with very large antenna.and it's apparent there that photosynthesis in wild type algae is saturating around 450micromoles of photons/m3/second. that’s
about one quarter of full sunlight intensity.in contrast, algae with no peripheral antenna complex such as cbs3 have higher rates ofphotosynthesis at high light intensities, whereas the intermediates actually do betterthat the very small antenna or the very large antenna. now these rates are expressed ona chlorophyll basis, and so they're somewhat marginalized by a small reduction in chlorophyll,but also on a cell basis we see the same trends, and that is algae with intermediate size antennahave higher rates of photosynthesis than algae with very small antenna or algae with verylarge antenna. does that convert into increase in biomass? and the answer here is yes. underlow light conditions, you can see here that the growth indicated by cell density of wildtype algae and algae with intermediate size
antenna, is virtually the same, whereas algaewith very small antenna are not growing well at all. under high light or saturating lightconditions, again the algae with the large antenna are doing better than wild type orthe algae with the small antenna. so these algae that i just described have mixed antennasso as light intensity changes through the day, it changes throughout seasons, it's aconstantly changing input that drives photosynthesis. so ideally we would like to have antenna thatcould self-adjust, and optimize their photosynthetic efficiency regardless of the light intensityduring the season, or during the day. and so we've developed a strategy which we canregulate the size of the antenna as a function of the light intensity in which the algaeare growing. so these are self-adjusting antennas.
and the strategy here is we start with algaethat have no capability of making chlorophyll b, we then introduce the gene called chlorophyll-aoxygenase that allows them to make chlorophyll b, but at the 5 prime end of the gene we adda 19 base pair light response element to that messenger rna. that light response elementis the binding site for a translational inhibitor called nab 1, that was identified by olafkruse's lab in germany. nab 1 will prevent the translation of the messenger rna to whichthe lre is fused. nab 1 in turn is regulated by light intensity. there is abundant andvery high levels of nab 1 under high light, and low levels of nab 1 under low light. sothe light regulation is translationally controlled by the nab 1 protein, and the model predictsthat under high light, nab 1 blocks translation
of the chlorophyll a oxygenase, chlorophyllb synthesis is inhibited the a/b ration goes up and the antenna size shrinks. the oppositecase is low light, and under those conditions, nab 1 levels are reduced, that allows thetranslation of the chlorophyll a oxygenase messenger rna, chlorophyll b synthesis goesup as well as antenna size, and it would be predicted that the cultures would grow wellunder low light conditions with big antennas provide an advantage. so those were actuallythe outcomes of this experiment, so in this particular experiment, we're looking at thegrowth of algae with different antenna sizes as well as capabilities to modulate the sizeof their antenna. so the blue line are wild type organisms, including a complemented wildtype with the cao or chlorophyll a oxygenase
gene but lacking the light responsive element.and you can see over the course of time that the a/b ratio, or the indicator of the antennasize does not change as culture density increases with time. that's in contrast to those withthe self-adjusting antenna. and so in the beginning when we do have a low inoculationor low cell numbers, there's high light intensity throughout the culture and the antenna aresmall. as the light intensity is diminished, the cell number increases and the antennasize increases and that's indicated by a reduction in the a/b ratio. so here we're actually lookingat the rate of photosynthesis, again, as a function of light intensity, for algae thathave self-adjusting antenna, those are the nab strains. algae with no chlorophyll b synthesisin vary small antenna, that's bcs3, and then
complemented wild type, and complemented wildtypes with a cao gene containing the light responsive element but a mutated light responsiveelement that would no long bind the nab protein, so a number of controls here. and what youcan see is that those algae that were indicated by the last figure to have the largest, thegreatest increases, or changes i should say, in antenna size, actually have here also thehighest rates of photosynthesis as a function of light intensity. three times greater thanthe wild type strains. so does that translate into increased biomass? and the answer isyes, and in fact we had a twofold increase in dry weight productivity as well as cellnumbers over the course of a 12 day cultivation under environmental conditions mimicking whatwould happen actually in a pond. and so this
approaches our objective of a threefold increaserequired to reduce the cost of producing fuel from 8 dollars to 3 dollars, so a simple 1gene transgenic trait that improves the efficiency of photosynthesis increases biomass productivitycan potentially help us to achieve our objective of reducing the price of fuels generated fromalgal biomass to levels that are competitive with petroleum. now very importantly, we'vealso demonstrated in separate experiments that these algae with engineered antenna sizeswill not outcompete wild type algae mixed cultures, and in fact they lose and wild typealgae win. and the reason for that, as i mentioned earlier, having a large antenna, even if wasteful,allows you to captures more light and exclude it form your competing organisms. so thisis an examples of a trait improvement that
actually reduces evolutionary fitness in thewild, but as a monoculture improves the overall yield of algal production. so in summary,what we've discussed today is the potential for algal biofuels becoming economically feasible.as we've discussed, we need to increase the yields by a factor of 3 over current productionrates, we need to have higher energy return on investment, ideally greater than 10, andwe need to reduce carbon emissions and enhance environmental services, in other words increasesustainability. there are some concerns about using gm technology, and perhaps the greatestconcern is actually weediness and invasive species that could potentially lead to ecosystemdisruption. but many of the traits that we’ll likely be engineered into algae are traitsthat will also reduce environmental fitness
and competition with other organisms, forexample, increasing the oil content is known in many cases to reduce the rate of cell division,and so there's not going probably be an evolutionary advantage to having higher oil content inthe wild. but to address concerns of course, it's always wise to be careful, and one approachis to introduce biocontainment traits into algae, some of those can be through traditionalapproaches such as mutagenesis, so traditional invalid mutations, those can potentially bestacked, introducing mutations that will prevent the capability of carrying out meiosis anddiploid production strains, and transfer of genes through mating, or the introductionof terminator gene technology that are under the control of xenobiotics that are presentin ponds but absent in the wild environment.
regulatory processes are in place at the presenttime, and the environmental protection agency currently has authority over field trialsand commercialization of gm algae, but depending on the trade, the usda as well as the fdamay also have regulatory approval processes. so in summary, there's great potential forimprove in productivity, producing high value coproducts from algae, increasing the sustainabilityof algae using genetic modifications, but you have to be careful, and we need to carryout the appropriate evaluations of field trials to be sure that the product that we generatethrough advanced technology not only improves the environment but are safe for the futureas well. thank you. so thank you and thank your dr sayre for joining us today. as i mentionedbefore, these slides will be made available
on the bioenergy technologies office website.please direct any questions to eere_biomass@ee.doe.gov. we ask that you please include “gm algaeâ€in the subject line of your email. thanks!